Imaging techniques
Techniques and the facilities where you can find them
Overview
Like X-ray imaging, neutron imaging is made possible by the fact that different elements have different attenuation coefficients.
Neutron imaging is based on the detection of the transmission of a neutron beam through an object. When this beam passes through, the object´s different components attenuate the neutron beam differently, depending on their composition. Hydrogen for example has a higher attenuation coefficient than lead or copper, which means that the signal detected for hydrogen will not be as strong as the signal detected for lead or copper. This allows us to produce accurate pictures of objects which give information about their structure.
Real-time imaging also allows to see movement within objects, such as oil distribution or water uptake.
The mechanisms of interaction of neutrons and X-rays with matter are extremely different from each other. Neutrons give a higher contrast for hydrogen containing materials such as water or oil, compared to X-rays, making neutron imaging the method of choice for many special industrial and physical applications. Today, resolutions as good as 25 µm can be achieved with both for Radiography and Tomography.
Neutron Imaging can have the unfortunate side-effect of leaving imaged samples radioactive if they contain appreciable levels of certain elements.
Classically, Neutron Imaging has been used for quality control purposes in industries that require precision machining such as aircraft or motor engineering. Today, one important application of neutron radiography is in testing the performance of fuel cells by imaging water or Hydrogen flow in the cells in situ. Neutron Imaging has also been used in the art world and in archeology to authenticate paintings and examine artifacts made of metal or stone.
Neutron Radiography
In neutron radiography (NR) we obtain 2D transmission images of objects revealing their inner structure. This can help to non-destructively detect cracks or pores or other material defects for example. With a neutron beam, a high contrast even between elements of low atomic number can be obtained. This property is useful for detection of hydrogen distribution in oil films in engines for example, or to study water uptake in plants or concrete.
Where to find this technique?
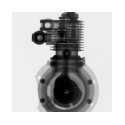
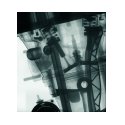
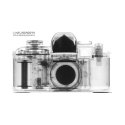
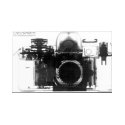
Neutron Tomography
Neutron tomography provides 3D data of an object from transmission data, measured by irradiating it from many different directions. Such a dataset can then be visualised in a volume rendering software showing the spatial distribution of materials in the sample. Virtual cuts through the object can be made in arbitrary directions and different materials can be assigned different colors allowing a clearer distinction of materials.
Tomographic imaging in a mathematical sense deals with reconstructing an image from its projections. Here the projection at a given angle represents the integral of the neutron absorption in the direction specified by that angle.
Where to find this technique?
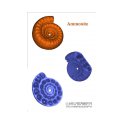
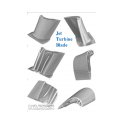
Real-time imaging
In real-time imaging the aim of the investigation is to follow a dynamic process in real time. This often requires the tuning of the scintillator and camera frame rate to meet the timing requirements of the process. The figure shows a time series of a water droplet on sand. The three frames are extracted from a sequence that was captured with 4 frames per second. The time between first and last image is 2.75s.
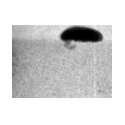
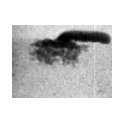
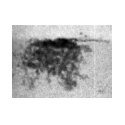
Stroboscopic imaging
Dynamic processes, whereby the same event is repeated cyclically, can benefit from stroboscopic imaging. A trigger signal from the process starts the data acquisition. If the process is fast, several images from the same state can be superimposed to improve the counting statistics.
Below are frames from a two-stroke engine running at 3000 rpm.
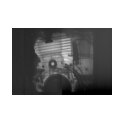
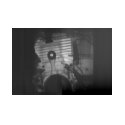
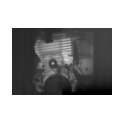
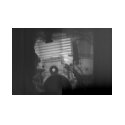
Energy-selective imaging
The energy selector allows investigations with a narrow banded neutron beam. This can be used
to study crystalline structures in materials. The figures below show a steel weld observed with
different neutron energies.
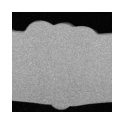
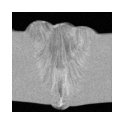
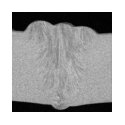
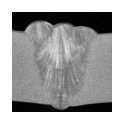
Neutron grating interferometry
The interferometer uses the wave behaviour of the neutron beam. In addition to the transmission image, this method also provides phase contrast and dark field image showing.
Further reading:
Neutron imaging – past, present and future by Juliette Savin